The Quantum Device Lab has been granted the project SuperMOOSE by IARPA, the US research funding agency. Coordinated by our group, the project aims to establish a connection between two error-corrected qubits, laying the foundation for future quantum computers. Our team collaborates with researchers from MIT, Forschungszentrum Jülich, Université de Sherbrooke in Canada and two industry partners, Zurich Instruments and Atlantic Quantum.
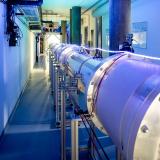
Researchers from our group have demonstrated that superconducting circuits separated by a cryogenic link spanning a distance of 30 metres can be much more strongly correlated with each other than is possible classically. This loophole-free Bell test firmly establishes non-locality is a resource in quantum information technology based on superconducting circuits.
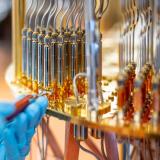
Operations on quantum computers, like on any technology, are inevitably subjected to errors, due to both external sources of interference and imperfections in their operation. But coping with quantum errors is particularly challenging. Writing in Nature, Sebastian Krinner and Nathan Lacroix, scientists at the Quantum Device Lab, together with their collaborators and working with colleagues in Canada and Germany, report a substantial advance towards fault-tolerant quantum computers. Using 17 physical qubits in a superconducting circuit, they demonstrate quantum error correction using the so-called surface code, which is known for its exceptionally high tolerance to errors.
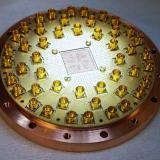
ETH Zurich and the Paul Scherrer Institute (PSI) establish a joint centre for the development of quantum computers. ETH Zurich provides 32 million francs for this centre, which will host around 30 researchers. Under the leadership of Andreas Wallraff and Jonathan Home they will pursue research in the two technology areas of superconducting circuits and ion traps. In the future, further groups working on related topics will complement the research centre. The Quantum Computing Hub will be located on the PSI campus in Villigen, Canton of Aargau. For this purpose, an existing building was converted specifically for research on quantum computers.

Quantum computers, which have the capability to outperform classical computers on specific computational tasks, heavily rely on high-performance two-qubit gates for the realization of quantum algorithms. In superconducting circuits, two-qubit gates are typically based on a transversal qubit-qubit coupling, implemented either by rf-control or the in-situ frequency tunability of computational qubits.
In this work, we demonstrate a novel approach using a tunable cross-Kerr-type ZZ interaction between two qubits which we realize with a flux-tunable coupler element.
This approach ensures direct control of the acquired conditional phase, without relying on excitation transfer or sideband transitions, and thus features an inherent resilience to leakage and cross talk, both major concerns in the light of recent progress towards full-scale quantum error correction. Additionally it innately enables the realization of continuous gate sets, highly beneficial for near-term variational quantum algorithms. A simple control paradigm and robust performance ensure easy scalability and high compatibility with existing platforms.