In the second round of ETH+ the Executive Board decided to financially support the initiative ETH Centre for Quantum Science and Technology. The planned Centre will pool the quantum research activities across the engineering and natural sciences at ETH as well as the Paul Scherrer Institute PSI to strengthen this field at the national level. There are also plans to establish a joint professorship with PSI for experimental quantum technology as well as a professorship for quantum information at the Department of Computer Science.
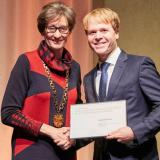
Congragulations to Sebastian Krinner for receiving the Lopez-Loreta Prize 2018, and with it a five-year research grant worth 1 million Euros.
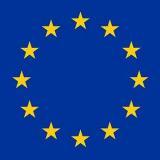
Ten international partners from academia and industry - including the Quantum Device Lab - will collaborate in a unique research endeavour to build a hybrid high-performance quantum computer. The new EU project OpenSuperQ (An Open Superconducting Quantum Computer), under the coordination of Saarland University, is part of the large-scale FET Flagship Initiative on Quantum Technologies. This unprecedented €1 billion initiative is funded by the European Commission and brings together experienced partners from across the EU.
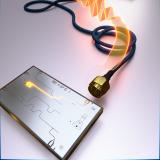
Sharing information over computer networks for private, business or science-related communication is part of our everyday lives. In the future, we may use protocols based on quantum physics to realize secure communication or to perform distributed quantum information processing exceeding the capabilities of classical computers and communication networks. In our work, we take a key step toward a future quantum network by realizing a fully deterministic quantum communication protocol between two remote superconducting quantum circuits. We accomplish this protocol by emitting a single, time-symmetric, itinerant microwave photon from one node of the network and absorb at another one to transmit a quantum bit of information and establish entanglement between two distant quantum nodes on-demand.
Nature 558, 264-267 (2018)
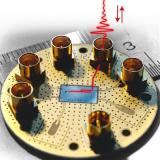
Information is often transmitted using electromagnetic radiation, the quantum units of which are photons. In the microwave regime, detecting single itinerant photons at the receiving end of a transmission channel is challenging since microwave photons possess 5 orders of magnitude less energy than their optical counterparts.
In this work, we show how to transfer the information content of a propagating photon into an excitation of a stationary qubit. By reading out the state of the latter, we acquire knowledge about the photon’s presence without destroying it. This ‘non-demolition’ aspect opens up new possibilities of detecting the photon in flight while allowing it to travel on towards another destination. Such schemes are potentially useful for realizing logic gates between propagating photons and for creating quantum networks.
Phys. Rev. X 8, 021003 (2018)